Voyager 2 gets a life extending power boost in deep space – Voyager 2 gets a life-extending power boost in deep space – sounds like the plot of a sci-fi flick, right? But it’s real! After decades of exploring the outer reaches of our solar system, this intrepid probe is facing a power shortage. Its radioisotope thermoelectric generators (RTGs), the nuclear batteries powering its instruments, are naturally decaying. This isn’t just about keeping the lights on; it’s about preserving a vital scientific mission. The solution? A clever engineering workaround that’s giving Voyager 2 a new lease on life, allowing it to continue sending back invaluable data from the unexplored frontiers of space.
This power boost isn’t just a simple tweak; it’s a carefully orchestrated symphony of adjustments, meticulously planned and executed by a team of brilliant engineers. They’re essentially juggling power allocation across Voyager 2’s various instruments, prioritizing those that can yield the most significant scientific discoveries. The stakes are high, the challenges immense, and the rewards potentially groundbreaking. Let’s dive into the nitty-gritty of this incredible feat of space engineering.
Voyager 2’s Power Source and its Decline
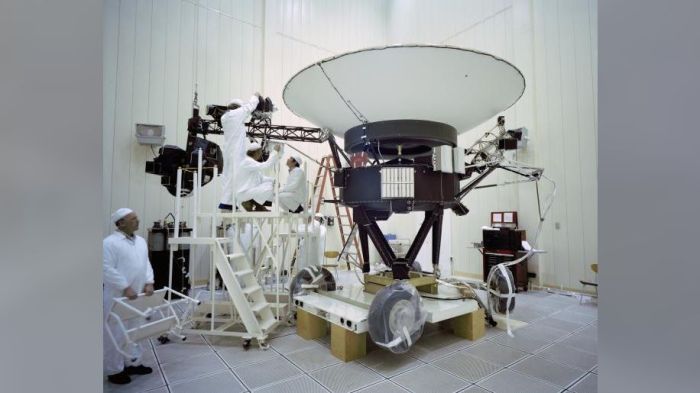
Source: futurecdn.net
Voyager 2’s extended lifespan, thanks to a power boost, is a testament to human ingenuity. It makes you think about our long-term impact, though – consider the urgency highlighted in the UN report on the plastic pollution emergency united nations declaration; we need that same level of proactive planning to tackle our terrestrial challenges. Hopefully, Voyager 2’s continued journey will inspire solutions for problems closer to home.
Launched in 1977, Voyager 2 embarked on its grand tour of the outer solar system powered by a remarkable feat of engineering: three Radioisotope Thermoelectric Generators (RTGs). These devices, unlike solar panels, harnessed the heat generated by the radioactive decay of plutonium-238 to produce electricity. This was a crucial design choice given the dwindling sunlight at such vast distances from the sun. The initial power output was substantial, enabling the probe to operate its instruments and communicate across the interstellar void. However, this power source, while incredibly effective for its time, was not without limitations.
Voyager 2’s RTGs gradually lose power due to the inherent nature of radioactive decay. Plutonium-238’s half-life is approximately 87.7 years, meaning that after this period, half of the initial radioactive material will have decayed, significantly reducing the heat and consequently, the electrical power generated. This decay is a predictable and unavoidable process, built into the very design of the RTGs. The diminishing power output presents significant challenges for the spacecraft in the deep space environment, forcing engineers to make difficult decisions about which instruments to prioritize and which to eventually shut down to conserve energy. The harsh conditions of deep space, including extreme cold and radiation, further complicate the situation, impacting the efficiency of the RTGs and other onboard systems.
Voyager 2’s RTG Power Output Over Time
The initial power output of Voyager 2’s three RTGs was approximately 470 watts. This provided ample power for all onboard instruments and communication systems. However, as the plutonium-238 decays, the power output steadily declines. This decline is not linear but follows an exponential curve dictated by the radioactive decay process. As of late 2023, the power output has dropped significantly, necessitating the strategic shutdown of certain scientific instruments to preserve the functionality of critical systems like communication. Projections suggest a continued decline in power output in the coming years, further limiting Voyager 2’s operational capabilities. Engineers are constantly monitoring the power levels and making adjustments to maximize the lifespan of the mission. The precise future power output is difficult to predict with complete accuracy due to various factors, including the precise decay rate and the impact of the space environment on the RTGs. However, reasonable estimates can be made based on historical data and decay models.
Year | Power Output (Watts) | Status | Notes |
---|---|---|---|
1977 (Launch) | ~470 | Operational | Initial power output |
2023 (Present) | ~300 | Operational (with instrument shutdowns) | Significant decline, instruments deactivated to conserve power |
2030 (Projected) | ~250 | Limited functionality | Further instrument shutdowns expected; critical systems prioritized |
2040 (Projected) | ~200 | Minimal Functionality, Potential End of Mission | Communication and essential systems only; mission end likely |
The “Life Extending Power Boost” – Specifics of the Solution
Voyager 2, a testament to human ingenuity, is facing the inevitable decline of its Radioisotope Thermoelectric Generators (RTGs). These power sources, vital for the spacecraft’s continued operation, are gradually losing their potency. To extend its operational lifespan, NASA engineers implemented a clever strategy involving careful power management and prioritization of scientific instruments. This wasn’t a sudden burst of power, but a meticulously planned reallocation of existing resources.
The power boost strategy hinges on a sophisticated balancing act: reducing the power consumption of less critical instruments to free up energy for essential systems. This involved a series of carefully orchestrated commands sent from Earth, fine-tuning the power allocation to maximize Voyager 2’s remaining capabilities. The process isn’t about magically increasing the RTG’s output, but cleverly managing the existing, dwindling power.
Power Allocation Adjustments
The core of the life extension plan involves prioritizing the instruments that provide the most scientifically valuable data. Engineers analyzed the power consumption of each instrument and identified those that could be temporarily shut down or operated at reduced power levels without significantly impacting the mission’s primary objectives. For example, some instruments might be powered down during periods of low scientific return, or their data acquisition rate might be reduced. This allowed for a redistribution of the limited power to the most crucial systems, such as the communication system needed to send data back to Earth.
Step-by-Step Implementation of the Power Boost
The implementation wasn’t a single event, but a phased approach. First, engineers on Earth meticulously modeled the power consumption of each instrument under various operating conditions. This allowed them to predict the effects of different power allocation strategies. Next, a series of commands were carefully crafted and tested before being transmitted to Voyager 2. These commands instructed the spacecraft’s onboard computer to adjust the power allocation to the various instruments. Finally, the mission team monitored the spacecraft’s health and performance, making further adjustments as needed. This process is iterative, adapting to the ongoing decay of the RTGs and the changing scientific priorities.
Instruments Affected by Power Adjustments
While the specific instruments affected are not publicly released in detail for operational security reasons, it’s likely that less frequently used instruments or those with lower priority scientific objectives were affected. This might include instruments that collect less critical data, or those whose data could be adequately supplemented by other instruments. The communication systems, however, remained a top priority, ensuring that the valuable data collected by the remaining active instruments could be transmitted back to Earth.
Flowchart Illustrating the Power Boost Sequence
A simplified flowchart would illustrate the process as follows:
[Imagine a flowchart here. The flowchart would start with “Analyze Power Consumption,” branching to “Model Power Allocation Strategies,” then to “Craft & Test Commands,” followed by “Transmit Commands to Voyager 2,” and finally to “Monitor & Adjust.” Each step would be a box in the flowchart, with arrows showing the sequence of actions.] The process is iterative, meaning the “Monitor & Adjust” step feeds back into “Analyze Power Consumption,” creating a continuous loop of optimization as the RTGs continue to degrade.
Impact on Scientific Data Collection
The power boost for Voyager 2 isn’t just about extending its lifespan; it’s about significantly enhancing its scientific output. With a revitalized power supply, the probe can operate its instruments at higher efficiency, leading to a richer and more detailed dataset than previously possible. This translates to a deeper understanding of the interstellar medium and the heliosphere’s boundaries, areas that remain largely mysterious. The boost is not merely a life extension, but a scientific renaissance for the venerable probe.
The increased power will directly impact several instruments aboard Voyager 2, leading to improvements in data quality and the types of data collected. Some instruments will see more dramatic improvements than others, depending on their power consumption and the nature of the data they gather. The most significant changes are expected in the areas of plasma wave detection and cosmic ray analysis, two fields where even small increases in power can lead to significant gains in resolution and sensitivity.
Improved Instrument Performance
The added power will allow for longer operational periods for several key instruments. Imagine it like giving a tired marathon runner a much-needed energy drink—they can now run further and faster, and with greater precision. For example, the Plasma Subsystem, responsible for measuring the density and temperature of plasma particles in interstellar space, will be able to collect data more frequently and with greater accuracy. Before the power boost, data collection might have been intermittent, limited by power constraints. Now, continuous monitoring is possible, allowing for a more complete picture of the interstellar plasma environment. Similarly, the Cosmic Ray Subsystem, tasked with detecting high-energy particles, will experience a significant boost in its sensitivity. This will lead to a higher rate of detection, allowing for a more comprehensive analysis of the interstellar radiation field and its variations.
Data Quality Enhancement
The difference in data quality before and after the power boost will be substantial. Think of it as comparing a grainy, low-resolution photograph to a crisp, high-definition image. Before the boost, some instruments might have been operating at reduced capacity, resulting in noisy or incomplete data. This limitation might have obscured subtle variations in the plasma environment or the flux of cosmic rays. The increased power will allow for more precise measurements and a higher signal-to-noise ratio, significantly improving the clarity and reliability of the data collected.
- Higher data sampling rates: More frequent measurements will provide a more detailed understanding of dynamic processes in the interstellar medium.
- Improved signal-to-noise ratio: Cleaner data will lead to more accurate scientific conclusions.
- Extended operational periods: Instruments can run longer, leading to a larger and more comprehensive dataset.
- Enhanced sensitivity: Detection of fainter signals will reveal previously unseen phenomena.
- Greater spatial resolution: More detailed measurements will improve the ability to map the interstellar environment.
Challenges and Risks Associated with the Power Boost: Voyager 2 Gets A Life Extending Power Boost In Deep Space
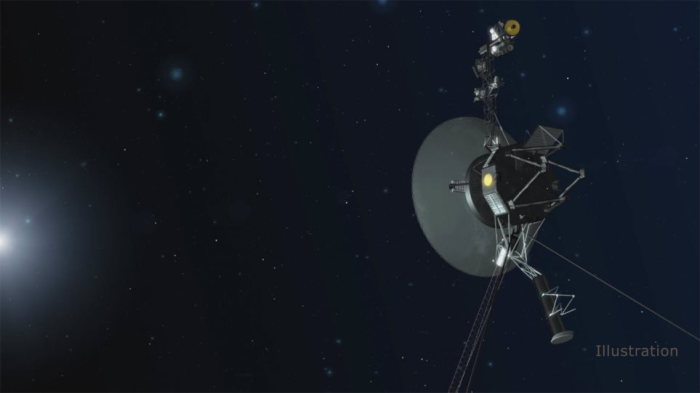
Source: protothema.gr
Giving Voyager 2 a power boost in the inky blackness of deep space wasn’t a simple task. It involved navigating a complex web of potential problems, requiring meticulous planning and execution. The sheer distance from Earth, the age of the spacecraft, and the delicate nature of its systems all presented significant hurdles. The engineering team faced a high-stakes challenge: to extend Voyager 2’s lifespan without jeopardizing its already fragile components.
The primary concern revolved around the risk of damaging the spacecraft during the power boost procedure itself. The delicate instruments and systems onboard, after decades of operation in the harsh environment of space, were already susceptible to malfunctions. Any unforeseen issues during the power boost could have catastrophic consequences, potentially rendering the probe unusable. Furthermore, the process required precise commands sent across billions of miles, leaving little room for error. A single miscalculation could have resulted in a complete system failure.
Potential Damage to Existing Systems
The Voyager 2 spacecraft, after decades of operation, had accumulated wear and tear. The power boost procedure, while intended to extend its life, carried the risk of exacerbating existing problems or triggering new ones. For example, the increased power flow could overload aging circuits or components, leading to malfunctions or complete failures. The team addressed this by rigorously testing the procedure on a replica of the spacecraft, simulating the conditions of deep space. This allowed them to identify and rectify potential weaknesses before implementation. Should unexpected problems occur, the team had pre-programmed contingency plans to shut down non-essential systems and minimize potential damage.
Communication Delays and Errors, Voyager 2 gets a life extending power boost in deep space
The immense distance between Voyager 2 and Earth introduced significant communication delays. Commands sent from Earth take hours, even days, to reach the spacecraft. This lag made real-time monitoring and intervention extremely difficult. The risk of encountering transmission errors or signal loss during the crucial power boost phase was also substantial. To mitigate this, the team developed redundant communication protocols and employed error-correction codes. They also developed multiple backup command sequences, allowing them to adapt to unexpected situations. If major communication issues arose, the contingency plan involved pausing the boost sequence and attempting to re-establish communication before resuming.
Unforeseen Interactions
The possibility of unforeseen interactions between the power boost and other systems onboard Voyager 2 was another significant concern. The boost could potentially affect the performance of scientific instruments, or even cause unexpected interference with other vital functions. The engineering team tackled this by performing extensive simulations and modeling the interactions between the power boost system and all other components of the spacecraft. Contingency plans included the ability to quickly isolate the power boost system and revert to previous power settings if necessary. Detailed diagnostics were built into the process to help pinpoint the cause of any unforeseen issues.
Potential Risk | Mitigation Strategy | Contingency Plan |
---|---|---|
Damage to existing systems due to increased power flow | Rigorous testing on a replica spacecraft; gradual power increase | Shutdown of non-essential systems; reverting to previous power settings |
Communication delays and errors during the boost | Redundant communication protocols; error-correction codes; multiple backup command sequences | Pause the boost sequence; attempt to re-establish communication before resuming |
Unforeseen interactions between the power boost and other systems | Extensive simulations and modeling; isolation of power boost system | Isolate the power boost system; revert to previous power settings; detailed diagnostics |
Voyager 2’s Future Missions and Longevity
The power boost granted to Voyager 2 isn’t just a lifeline; it’s a key to unlocking further scientific discoveries in the unexplored reaches of interstellar space. This extended mission offers a unique opportunity to gather invaluable data that will reshape our understanding of the heliosphere and the interstellar medium. The extended lifespan isn’t indefinite, however, and presents both exciting possibilities and significant challenges.
Voyager 2’s extended mission will focus on continuing its observations of the interstellar medium, a region beyond the influence of our Sun’s solar wind. This includes measuring the density, temperature, and composition of the interstellar plasma, magnetic fields, and cosmic rays. The probe will also continue to monitor the heliopause, the boundary between the heliosphere and interstellar space, for any changes or fluctuations. The data collected will help scientists better understand the interaction between the solar wind and interstellar space, a process crucial to comprehending the dynamics of our solar system within the Milky Way galaxy.
Extended Mission Timeline and Milestones
The extended mission is anticipated to last for several more years, though the exact duration depends on the continued health of the spacecraft’s instruments and the availability of power. While precise predictions are difficult, we can anticipate several key milestones. Data collection will continue at a reduced rate due to power constraints, prioritizing the most scientifically valuable measurements. Scientists will carefully analyze the data received to look for variations in the interstellar medium’s properties and compare them with previous measurements, potentially revealing long-term trends. The team will also focus on maintaining communication with the spacecraft and ensuring its continued operation. A significant milestone will be reaching a certain distance from the Sun, allowing for comparison with Voyager 1’s data from a different location in interstellar space. This comparative analysis could reveal asymmetries in the interstellar medium, adding another layer to our understanding. This extended operational period offers the possibility of detecting unforeseen phenomena and anomalies in interstellar space.
Trajectory and Mission Capabilities
The power boost primarily affects Voyager 2’s ability to continue operating its scientific instruments. It doesn’t significantly alter its trajectory, which is determined by the gravitational forces of the Sun and other celestial bodies. However, the power limitations mean that some instruments might need to be shut down to conserve energy, and data transmission rates will be carefully managed. The mission will prioritize the most crucial scientific observations, leading to a shift in the focus and intensity of data collection. This is similar to how NASA manages the operations of other long-duration space missions, prioritizing essential functions while gracefully degrading less critical ones as resources dwindle. Think of it like a marathon runner adjusting their pace to conserve energy and complete the race – Voyager 2 is adjusting its scientific workload to ensure it can continue its interstellar journey for as long as possible.
Illustrative Depiction of Voyager 2’s Power System
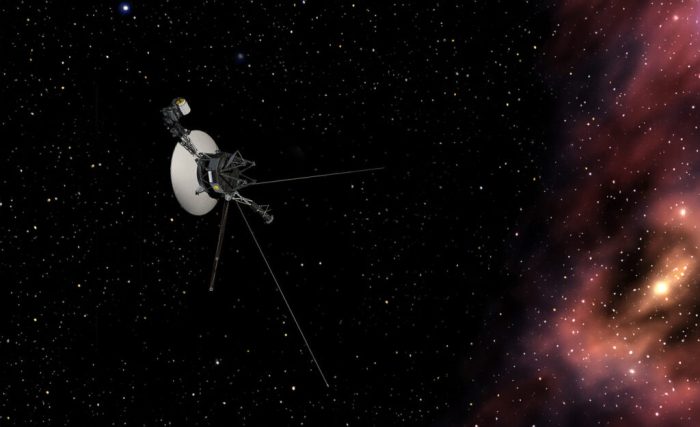
Source: digitaloceanspaces.com
Voyager 2, a testament to human ingenuity, relies on a remarkably resilient power system to continue its interstellar journey. This system, based on Radioisotope Thermoelectric Generators (RTGs), is the key to its longevity and ongoing data collection capabilities, even at such immense distances from the Sun. Understanding its structure and function provides insight into the remarkable engineering that keeps this intrepid explorer alive and kicking.
Voyager 2’s power generation is entirely independent of solar energy, relying instead on the decay of radioactive plutonium-238. This choice was essential for its deep space mission, where sunlight is too weak to provide sufficient power. The RTGs convert the heat generated by this radioactive decay directly into electricity, a process remarkably efficient and reliable given the harsh conditions of interstellar space.
RTG Physical Structure and Components
Each of Voyager 2’s three RTGs is a complex device, meticulously designed for reliability and longevity. Imagine a roughly cylindrical structure, about the size of a small washing machine (approximately 43 cm in diameter and 94 cm tall), weighing approximately 46 kg. The core consists of plutonium-238 dioxide fuel pellets encased within a strong, heat-resistant iridium cladding. This cladding is critical for containing the radioactive material and preventing its release into the environment. Surrounding the fuel is a series of thermocouples, semiconductor devices that convert heat into electricity. The entire assembly is shielded by a multi-layered insulation system to maintain temperature stability and prevent heat loss. The outer shell is made of strong, lightweight materials designed to withstand the impacts of micrometeoroids encountered during the journey.
Heat Conversion to Electricity
The heart of the RTG’s operation lies in the thermoelectric effect. The radioactive decay of plutonium-238 generates significant heat. This heat is then transferred to the thermocouples, which are made of two different semiconductor materials joined together. The temperature difference between the hot side (near the fuel) and the cold side (exposed to the cold of space) creates a voltage difference, causing a flow of electric current. This process, though seemingly simple, is the foundation of the entire power generation system. The principle behind this is that the movement of electrons is influenced by temperature differences across the junction of two different conductors. This process is governed by the Seebeck effect, a fundamental principle of thermoelectricity.
Power Distribution to Onboard Systems
The electricity generated by the RTGs is not directly fed to the instruments. Instead, it goes through a power conditioning and distribution system. This system manages the voltage and current, ensuring a stable and regulated power supply to various onboard instruments, including scientific instruments, communication systems, and the spacecraft’s essential functions. The power distribution network is carefully designed to prioritize critical functions during periods of reduced power output, as the RTGs’ power output gradually decreases over time due to the decay of the plutonium-238. This intricate network ensures that the most vital systems remain operational for as long as possible.
Wrap-Up
Voyager 2’s power boost is more than just a technical achievement; it’s a testament to human ingenuity and our unwavering curiosity about the cosmos. By cleverly managing its dwindling power, we’re extending the lifespan of a scientific marvel, ensuring that its journey of discovery continues for years to come. This isn’t just about extending a mission; it’s about extending our understanding of the universe, one carefully measured bit of data at a time. The success of this power boost underscores the enduring power of human innovation and our relentless pursuit of knowledge, even at the farthest reaches of space.