The massive batteries hidden beneath your feet—it sounds like science fiction, right? But the reality is far more fascinating. Imagine a future where gigawatt-scale battery systems, tucked away underground, silently power our cities and stabilize the energy grid. This isn’t just a pipe dream; engineers and scientists are already exploring the feasibility of large-scale underground energy storage, tackling challenges like heat dissipation and geological considerations to unlock a new era of clean, reliable power. This exploration delves into the technical marvels, economic implications, and societal shifts that could be triggered by this revolutionary approach to energy storage.
We’ll unpack the different types of batteries suitable for subterranean deployment, comparing their energy density, lifespan, and environmental impact. Beyond the literal, we’ll explore the metaphorical meaning of “hidden batteries,” examining how untapped resources and potential innovations across various sectors mirror this concept. Finally, we’ll look at the future of energy storage, considering advancements beyond large-scale batteries and their role in shaping a sustainable energy landscape.
The Literal Meaning
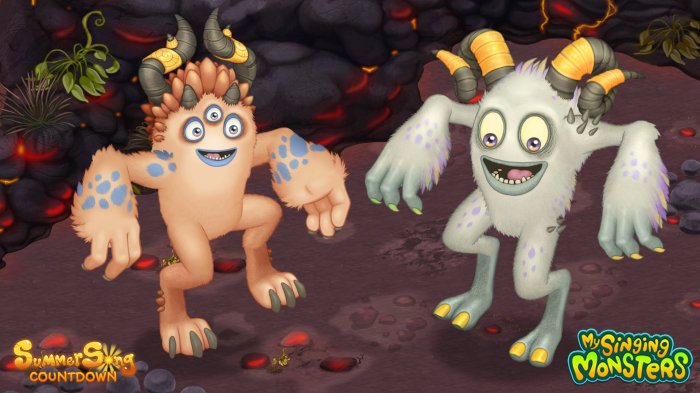
Source: twimg.com
Imagine a city humming with clean energy, its power silently stored beneath the very streets you walk on. This isn’t science fiction; it’s the emerging reality of large-scale underground energy storage. This technology tackles the intermittent nature of renewable energy sources like solar and wind, ensuring a stable and reliable power supply.
Underground Battery Technologies
Several battery technologies are suitable for subterranean deployment, each with its own advantages and disadvantages. Flow batteries, for example, excel in their long lifespan and scalability. These systems store energy in liquid electrolytes, pumped between tanks for charging and discharging. Their modular design makes them ideal for expansion and adapting to fluctuating energy demands. Another promising technology is lithium-ion batteries, already prevalent in consumer electronics, adapted for large-scale underground installations. Their high energy density allows for significant power storage in a relatively small footprint, though thermal management becomes a critical concern in large-scale deployments. Finally, pumped hydro storage (PHS) is a well-established technology where excess energy pumps water uphill, and the release of water generates electricity during peak demand. While not strictly a battery, PHS shares the function of energy storage, especially suited to underground caverns.
Engineering Challenges of Underground Battery Systems
Building and maintaining underground battery systems presents significant engineering challenges. The most immediate concern is thermal management. Large battery arrays generate substantial heat, requiring sophisticated cooling systems to prevent overheating and potential hazards. The underground environment itself presents unique challenges: water ingress, seismic activity, and the need for robust ventilation systems are all critical considerations. Access and maintenance are also complex, necessitating careful planning of infrastructure and potentially the use of robotics for inspection and repair. Ensuring structural integrity of the underground facility, resistant to ground shifts and potential flooding, is paramount.
Environmental Impact Assessment
Before construction, a comprehensive environmental impact assessment is crucial. This process involves evaluating potential impacts on groundwater, soil quality, and local ecosystems. The assessment considers the entire lifecycle of the battery system, from material extraction and manufacturing to decommissioning and waste management. Potential noise and light pollution during construction need to be addressed. Mitigation strategies, such as using sustainable materials and employing best practices for minimizing environmental disruption, are essential components of the assessment. Regulatory approvals are typically required, ensuring adherence to environmental protection laws and regulations. This often includes public consultation to address community concerns.
Cost-Effectiveness of Underground Battery Storage
The cost-effectiveness of underground batteries compared to other energy storage solutions, such as pumped hydro or compressed air energy storage (CAES), depends on several factors including site-specific conditions, technology choices, and energy prices. While the initial capital investment for underground battery systems can be high, the long lifespan and scalability can lead to lower long-term costs. The cost comparison should also account for land use, transmission losses, and environmental impacts. For instance, while pumped hydro might offer lower initial costs, it requires significant land and water resources, potentially limiting its applicability. Underground battery storage can offer a more compact and geographically flexible solution, especially in densely populated areas with limited surface space.
Comparison of Battery Chemistries for Underground Use
Battery Type | Energy Density (Wh/kg) | Lifespan (cycles) | Environmental Impact |
---|---|---|---|
Lithium-ion (Li-ion) | 150-250 | 5000-10000 | Moderate (mining, recycling challenges) |
Flow Batteries (e.g., vanadium redox) | 25-50 | 20000+ | Lower (less critical raw materials) |
Sodium-ion (Na-ion) | 100-150 | 5000-8000 | Lower (abundant materials) |
Zinc-air | 100-150 | 2000-5000 | Lower (relatively benign materials) |
Metaphorical Interpretation: The Massive Batteries Hidden Beneath Your Feet
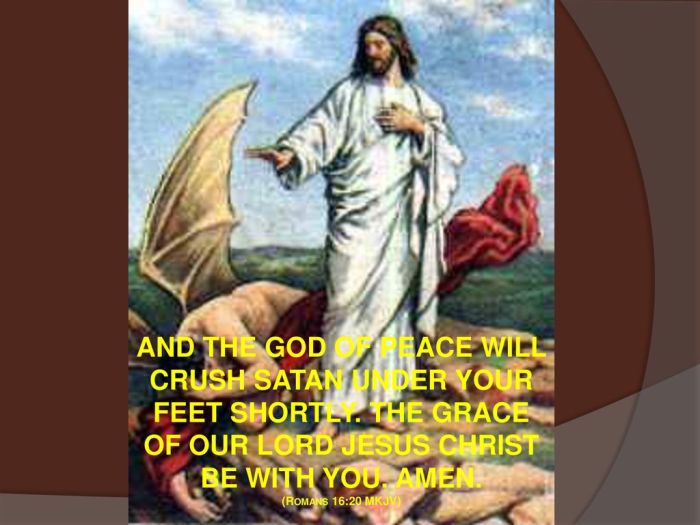
Source: slideplayer.com
The idea of “massive batteries hidden beneath our feet” transcends its literal meaning, serving as a potent metaphor for the vast, untapped potential residing within our world and ourselves. This hidden potential represents a wealth of resources and capabilities waiting to be discovered and harnessed, driving societal advancements and reshaping our future. The metaphor speaks to the often-overlooked possibilities that lie dormant, waiting for the right spark to ignite their transformative power.
The concept of hidden potential resonates across numerous fields. It’s a concept that underscores the importance of exploration and innovation, urging us to look beyond the readily apparent and to dig deeper to uncover the resources that can propel us forward. This metaphor encourages a mindset of persistent investigation and a belief in the possibility of breakthroughs, even in seemingly barren landscapes.
Hidden Potential in Renewable Energy
The quest for sustainable energy sources is a prime example of uncovering hidden potential. For centuries, humanity relied heavily on fossil fuels, seemingly the only viable energy source. However, the limitations and environmental consequences of fossil fuels spurred a search for alternatives. This led to the exploration and development of renewable energy sources like solar, wind, and geothermal energy – resources that were once considered insignificant or impractical but now represent a powerful force in the global energy transition. The seemingly endless potential of the sun, wind, and earth’s core were hidden until we developed the technology to harness them.
Technological Innovation Fueled by Hidden Resources
Technological advancements often stem from the discovery and utilization of previously overlooked resources or capabilities. The development of the silicon chip, for instance, revolutionized computing and countless other industries. Silicon, a relatively abundant element, was transformed from a commonplace material into the foundation of modern technology, demonstrating the transformative power of recognizing and exploiting hidden potential. Similarly, the internet itself was built upon the previously underutilized potential of interconnected computer networks, revealing a hidden potential for global communication and information sharing.
Uncovering Hidden Human Capabilities
The metaphor of hidden batteries also applies to human potential. Many individuals possess untapped skills, talents, and abilities that remain dormant due to lack of opportunity, support, or self-belief. The realization and cultivation of these hidden capabilities can lead to significant personal and societal advancements. Consider the countless individuals who have overcome adversity to achieve extraordinary feats, showcasing the immense power residing within the human spirit – a hidden battery waiting to be charged.
Visual Representation of Unearthed Potential
Imagine a vast, seemingly barren landscape, cracked earth revealing only dust and rock. As excavators begin to dig deeper, they unearth a network of glowing, interconnected energy cells, radiating light and power. These cells represent the hidden potential, initially obscured but now revealed and ready to be harnessed, transforming the barren landscape into a vibrant, productive environment. The initial impression of emptiness is replaced by a stunning display of previously hidden resources.
Potential “Hidden Batteries” Across Sectors
The following list identifies potential “hidden batteries” in various sectors and their potential impact:
These examples highlight the significant impact that identifying and harnessing hidden potential can have on various aspects of society and technology. Further exploration and investment in these areas promise even greater advancements in the years to come.
Ever think about the massive batteries hidden beneath your feet – the planet’s geothermal energy? Harnessing that power is crucial, especially considering the economic devastation predicted by experts; check out this article on the looming El Niño could cost the world trillions of dollars , highlighting the urgent need for sustainable alternatives. Investing in geothermal energy could be a game-changer, mitigating the financial fallout from climate change and tapping into the massive batteries beneath our feet.
- Ocean Energy: Harnessing the power of ocean currents and waves for electricity generation. Potential impact: Significant reduction in reliance on fossil fuels, creation of new industries.
- Data Analytics: Uncovering hidden insights from vast datasets to improve decision-making in various sectors. Potential impact: Improved healthcare, optimized resource management, more efficient businesses.
- Biotechnology: Exploring the potential of biological systems to create new materials, medicines, and technologies. Potential impact: Development of life-saving drugs, sustainable materials, improved agricultural practices.
- Human Capital Development: Investing in education and training to unlock the potential of individuals and communities. Potential impact: Increased productivity, economic growth, social progress.
The Technological Feasibility
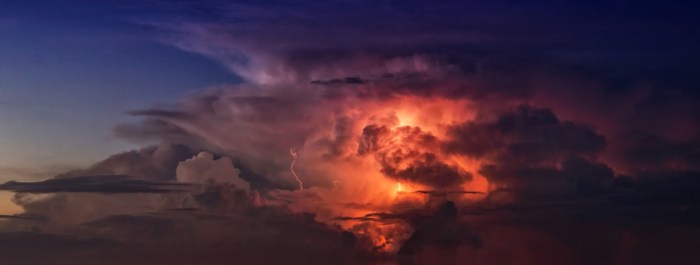
Source: nanjones.com
Building a large-scale underground battery network presents a significant technological challenge, requiring innovative solutions to overcome inherent limitations and ensure safety and efficiency. This undertaking necessitates a careful consideration of various factors, from heat management to geological suitability and emergency preparedness.
The sheer scale of such a project demands a multi-faceted approach, addressing not only the technological hurdles but also the logistical and economic considerations. The potential benefits, however, – enhanced grid stability, increased renewable energy integration, and improved energy security – are substantial enough to warrant the considerable investment and effort required.
Heat Dissipation and Safety Measures
One of the primary concerns in underground battery installations is heat management. Batteries generate heat during charging and discharging cycles, and this heat needs to be effectively dissipated to prevent thermal runaway, a dangerous condition that can lead to fires or explosions. Solutions include implementing advanced cooling systems, such as liquid cooling or air-cooling systems with optimized airflow, and selecting battery chemistries with inherently lower heat generation rates. Furthermore, robust fire suppression systems, including early detection mechanisms and specialized extinguishing agents, are essential safety components. The design must also incorporate features to mitigate the risk of gas buildup, a potential hazard associated with some battery chemistries.
Geological Considerations and Infrastructure Design
The geological characteristics of the chosen location significantly influence the design and implementation of an underground battery network. Rock type, water table levels, and seismic activity all play crucial roles. For example, stable bedrock formations offer superior structural support and better insulation compared to loose soil or unconsolidated sediments. The presence of groundwater necessitates careful waterproofing and drainage strategies to protect the batteries from moisture damage. In seismically active regions, the design must account for earthquake resistance and potential ground displacement. The excavation methods, the structural integrity of the underground chambers, and the overall layout of the network will all be dictated by the geological context.
Safety Protocols and Emergency Response
Comprehensive safety protocols and emergency response plans are crucial for ensuring the safe operation of an underground battery facility. These plans must include detailed procedures for handling various potential hazards, such as fires, gas leaks, and equipment malfunctions. Regular safety inspections, staff training, and robust monitoring systems are essential for early detection and prevention of incidents. The emergency response plan should Artikel evacuation procedures, communication protocols, and the coordination of emergency services. The design should also incorporate features that facilitate safe access and egress in case of an emergency, such as multiple escape routes and emergency lighting.
Building and Maintaining an Underground Battery Storage System
The process of building and maintaining an underground battery storage system is complex and involves multiple stages. Effective project management and rigorous quality control are essential throughout the entire lifecycle of the system.
- Site Selection and Geological Survey: Thorough geological surveys and risk assessments are conducted to identify suitable locations and mitigate potential geological hazards.
- Design and Engineering: Detailed engineering designs are developed, incorporating specifications for the battery systems, cooling systems, safety features, and access infrastructure.
- Construction and Installation: The underground chambers are excavated, and the battery systems, cooling systems, and safety equipment are installed and tested.
- Commissioning and Testing: Rigorous testing and commissioning procedures are followed to ensure the system’s functionality and safety before operation.
- Operation and Monitoring: Continuous monitoring of the system’s performance, temperature, and other critical parameters is carried out to ensure optimal operation and early detection of potential issues.
- Maintenance and Upgrades: Regular maintenance and upgrades are conducted to ensure the long-term reliability and safety of the system.
Societal and Economic Implications
The widespread adoption of underground energy storage, while promising a cleaner and more resilient energy future, presents a complex tapestry of societal and economic consequences. Its impact extends far beyond the technical realm, influencing energy markets, employment landscapes, and social equity. Understanding these implications is crucial for responsible development and deployment of this transformative technology.
The potential economic benefits are substantial. Reduced reliance on volatile fossil fuel markets could lead to greater energy independence and price stability. The creation of a robust underground energy storage infrastructure would stimulate economic growth, generating jobs in manufacturing, installation, maintenance, and related sectors. Moreover, the increased efficiency and reliability of the energy grid could reduce transmission losses and improve overall productivity. However, the initial investment costs associated with building this infrastructure are significant, potentially creating a barrier to entry for smaller companies and developing nations. The long-term economic viability hinges on factors such as technological advancements, government incentives, and the overall rate of adoption.
Economic Benefits and Drawbacks of Widespread Adoption
Widespread adoption of underground battery technology offers significant economic advantages, primarily through increased energy security and price stability. Reduced reliance on fluctuating fossil fuel prices would benefit both consumers and businesses. Furthermore, the creation of a new infrastructure would generate substantial employment opportunities across various sectors. However, the high upfront capital costs could hinder widespread adoption, particularly for smaller energy providers or developing countries. A detailed cost-benefit analysis, considering factors like lifespan, maintenance, and potential environmental impacts, is crucial for effective policy-making. For example, the successful implementation of similar large-scale infrastructure projects, such as high-speed rail networks, demonstrates that careful planning and long-term investment can yield substantial economic returns.
Impact on the Energy Market and Sustainable Energy Future
Underground energy storage is poised to play a pivotal role in a sustainable energy future by enabling greater integration of renewable energy sources like solar and wind power. These sources are inherently intermittent, and large-scale energy storage is crucial for ensuring a reliable and consistent energy supply. Underground batteries can act as buffers, storing excess energy generated during peak production periods and releasing it during periods of low generation or high demand. This helps to stabilize the grid, reducing the need for fossil fuel peaker plants and lowering carbon emissions. The shift towards a decentralized energy system, enabled by widespread adoption of this technology, would empower local communities and enhance energy resilience. Consider the example of California, which faces frequent power outages during heatwaves: widespread underground energy storage could significantly improve grid stability and reliability.
Social Implications and Accessibility
Compared to other energy storage solutions, such as pumped hydro storage or large-scale battery farms, underground energy storage offers potential advantages in terms of land use and visual impact. Pumped hydro, for example, requires significant land area and can alter local ecosystems. Large-scale battery farms, while more compact, can still raise environmental concerns related to battery material sourcing and disposal. Underground storage minimizes these impacts. However, the equitable distribution of this technology is crucial. Ensuring that the benefits are not concentrated in wealthy communities or regions is essential. Policy interventions may be needed to promote access to this technology in underserved areas, ensuring that all communities can benefit from a more reliable and affordable energy supply. For instance, targeted subsidies or tax breaks could incentivize the deployment of underground energy storage systems in low-income communities.
Effects on Employment and the Workforce
The development and deployment of underground energy storage will create a wide range of employment opportunities. These include jobs in engineering, manufacturing, installation, maintenance, and operations. However, it’s important to anticipate the potential displacement of workers in traditional fossil fuel industries. Retraining and upskilling programs will be crucial to ensure a smooth transition for these workers. Furthermore, the demand for specialized skills in areas like geotechnical engineering and battery management systems will increase, necessitating investment in education and training programs to meet this growing need. The transition, much like the shift from agrarian economies to industrial ones, presents challenges but also significant opportunities for economic diversification and growth.
Necessary Policy Changes to Facilitate Adoption, The massive batteries hidden beneath your feet
Several policy changes are necessary to accelerate the adoption of underground energy storage technology. These include streamlining permitting processes, providing financial incentives through tax credits or subsidies, and establishing clear safety and environmental regulations. Investing in research and development to improve the efficiency and cost-effectiveness of the technology is also crucial. Standardization of battery technologies and interoperability standards would facilitate wider adoption and reduce market fragmentation. Furthermore, regulatory frameworks should address issues related to land ownership, access rights, and environmental impact assessments to ensure a smooth and efficient deployment process. Examples of successful policy interventions include those used to promote renewable energy development, such as feed-in tariffs and renewable portfolio standards, which can be adapted and applied to underground energy storage.
The Future of Energy Storage
Forget the massive batteries hidden beneath our feet – that’s just the beginning. The future of energy storage is a vibrant tapestry woven from a multitude of innovative technologies, each vying for a place in a cleaner, more secure energy landscape. We’re moving beyond simply storing energy; we’re reimagining how we generate, distribute, and consume it.
The current reliance on lithium-ion batteries, while transformative, presents limitations in terms of scalability, sustainability, and raw material availability. The quest for better energy storage solutions is driven by the urgent need to address climate change and ensure reliable energy access for a growing global population. This necessitates a multi-pronged approach, exploring diverse technologies and addressing the challenges inherent in their development and deployment.
Advanced Battery Chemistries
Beyond lithium-ion, several promising battery chemistries are emerging. Solid-state batteries, for example, offer the potential for higher energy density, improved safety, and longer lifespan compared to their liquid-electrolyte counterparts. Solid-state technology eliminates the flammable liquid electrolytes, reducing the risk of fire and improving overall safety. Companies like Solid Power and QuantumScape are leading the charge in this area, although widespread commercialization remains a few years away. Meanwhile, research into sodium-ion batteries, leveraging the abundance and lower cost of sodium, is gaining traction as a more sustainable alternative to lithium-ion. These batteries, while currently less energy-dense, offer a compelling path towards more affordable and environmentally friendly energy storage solutions.
Beyond Batteries: Alternative Energy Storage Technologies
The pursuit of efficient energy storage extends far beyond batteries. Pumped hydro storage, a mature technology, involves pumping water uphill during off-peak hours and releasing it to generate electricity during peak demand. While geographically limited, it remains a significant player in large-scale energy storage. Compressed air energy storage (CAES) offers another approach, storing energy as compressed air in underground caverns or depleted gas fields. This technology is particularly suited for balancing intermittent renewable energy sources like wind and solar. Furthermore, thermal energy storage, using molten salts or other materials to store heat or cold, is gaining prominence in concentrated solar power plants and other applications requiring long-duration energy storage. Flywheel energy storage, utilizing the kinetic energy of a rapidly spinning rotor, offers fast response times and high efficiency, making it suitable for grid stabilization and other applications requiring quick energy release.
Challenges and Opportunities in Energy Storage Development
The development and deployment of advanced energy storage technologies face significant challenges. High initial costs, limited scalability, and the need for improved energy density and lifespan are key obstacles. However, substantial opportunities exist for innovation and investment. Government policies promoting renewable energy integration and energy storage deployment play a crucial role in driving technological advancements. Collaboration between research institutions, industry, and policymakers is essential to overcome technical hurdles and accelerate the commercialization of next-generation energy storage solutions.
A Timeline of Energy Storage Milestones
The development of energy storage technology has been a journey marked by both breakthroughs and setbacks. A timeline illustrates this evolution:
The following bullet points illustrate key milestones, highlighting both successes and failures in the development of energy storage technology:
- Early 19th Century: Early experiments with electrochemical cells lay the groundwork for future battery technologies. Many early attempts suffered from short lifespans and low energy density.
- Late 19th Century – Early 20th Century: Lead-acid batteries emerge as a commercially viable technology, powering early automobiles and other applications. However, their relatively low energy density limits their widespread adoption.
- Mid-20th Century: Development of nickel-cadmium and nickel-metal hydride batteries expands the range of applications for rechargeable batteries. However, concerns about toxicity and environmental impact emerge.
- Late 20th Century – Present: Lithium-ion batteries revolutionize portable electronics and begin to penetrate the electric vehicle and grid-scale energy storage markets. Challenges remain in terms of raw material sourcing, cost, and safety.
- Present – Future: Research and development focus on advanced battery chemistries (solid-state, sodium-ion), alternative storage technologies (pumped hydro, CAES, thermal storage), and improved energy management systems.
Final Summary
The idea of massive batteries hidden beneath our feet might seem futuristic, but the potential benefits are undeniably compelling. From stabilizing the energy grid powered by renewables to mitigating climate change, underground energy storage represents a significant leap forward in our quest for a sustainable energy future. While challenges remain—from technological hurdles to economic considerations—the potential rewards of unlocking this hidden power are too significant to ignore. The journey towards realizing this vision will require innovative engineering, strategic policy changes, and a collective commitment to a cleaner, more resilient energy system. The future of energy, it seems, may lie beneath our feet.